With the advent of the information technology revolution, the integrated circuit industry has developed rapidly. The increasing integration density of electronic systems leads to higher power density and greater heat generation from electronic components and the system as a whole. Therefore, effective electronic packaging must address the heat dissipation issues of electronic systems.
In this context, ceramic substrates, with their excellent heat dissipation performance, have seen a rapid surge in market demand. Particularly for aluminum nitride (AlN) ceramic substrates, despite their significantly higher prices compared to other substrates, they remain in short supply, even to the point of being "hard to find." Why is this?
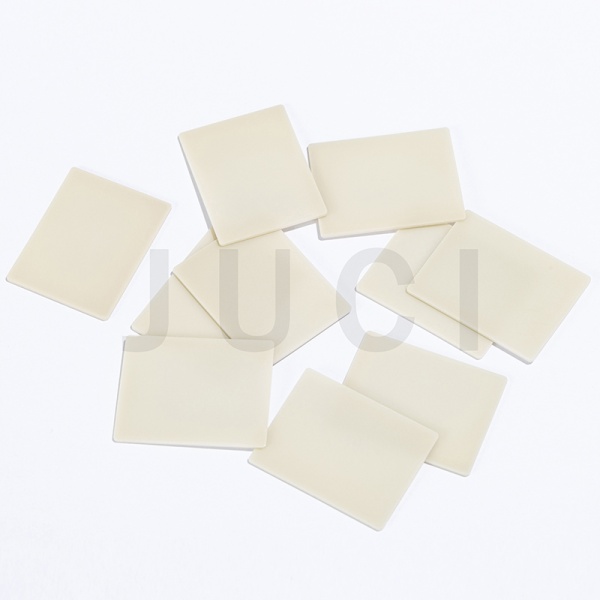
The reasons are simple. The author believes there are three main points:①Superior performance, worth the price②Challenging production process③Rapid market growth.
Today, we will delve deeper into these three aspects to better understand AlN ceramic substrates.
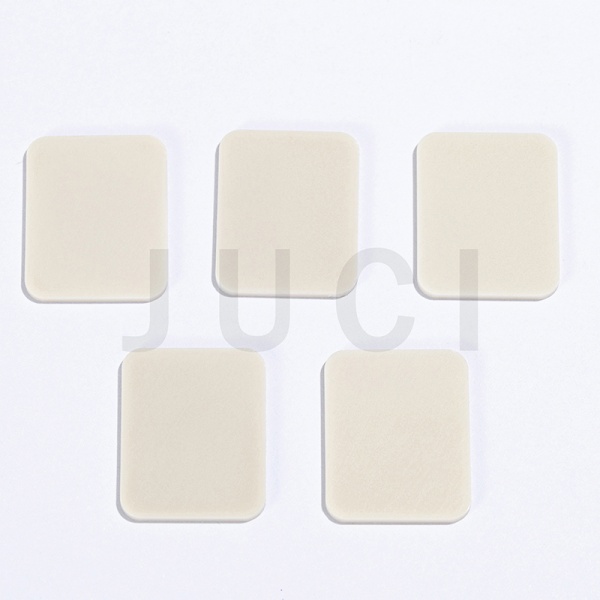
01 Outstanding Thermal Conductivity
First, ceramic packaging substrates primarily rely on the material's high thermal conductivity to transfer heat away from the chip (heat source) and facilitate heat exchange with the external environment. For power semiconductor devices, packaging substrates must meet the following requirements:
High thermal conductivity to meet heat dissipation needs.
Excellent heat resistance to withstand high-temperature applications (above 200°C).
Matched coefficient of thermal expansion (CTE) with chip materials to reduce thermal stress in packaging.
Low dielectric constant for high-frequency performance, reducing signal transmission delay and improving speed.
High mechanical strength to meet the mechanical performance requirements during packaging and application.
Good corrosion resistance to withstand strong acids, alkalis, boiling water, organic solvents, etc.
Dene structure to meet the hermetic sealing requirements of electronic devices.
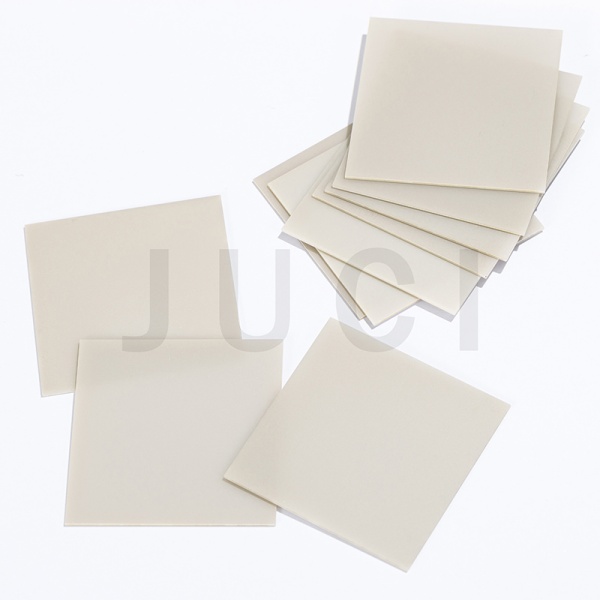
So, how does aluminum nitride perform? As a ceramic substrate material, AlN ceramic parts boast high thermal conductivity, high strength, high resistivity, low density, low dielectric constant, non-toxicity, and a CTE matching Si. These properties make it one of the most promising high temperature ceramic materials.
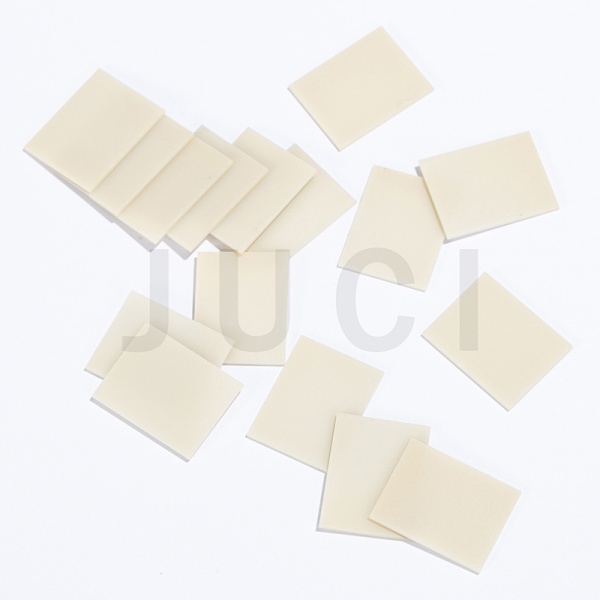
02 Complex and Tedious Production Process
The production of AlN ceramic substrates is highly complex and tedious, primarily reflected in two aspects: the preparation of high-end AlN powder and the fabrication of the substrates. Let’s explore these two areas separately.
1. Aluminum Nitride Powder
The quality of almost all industrial ceramic products is heavily influenced by the raw material quality, and AlN ceramic substrates are no exception.
(1) Powder Preparation Methods
As a high-performance powder material, researchers worldwide continue to innovate to address technical challenges in existing processes while exploring new, more efficient preparation methods. Currently, the most widely used industrial methods are carbothermal reduction and direct nitridation, which are mature, simple, and yield high-quality products.
(2) Multiple Factors Affect Powder Performance
The performance of AlN ceramic products depends directly on the characteristics of the raw powder, particularly its most valuable property—thermal conductivity. Key factors influencing thermal conductivity include:oxygen and other impurity content,sintering density,microstructure.These factors are reflected in the AlN powder’s purity, particle size, and shape.
(3) Hydrolysis Sensitivity and the Need for Modification
Despite its excellent properties, AlN powder has a major drawback: it is highly prone to hydrolysis. In humid environments, it readily reacts with hydroxyl groups in water to form aluminum hydroxide, creating an alumina layer on the surface. This layer dissolves oxygen into the lattice, reducing thermal conductivity and altering physicochemical properties, complicating its application.
The current solution is to coat the aluminum nitride particles with a substance that isolates them from water, preventing hydrolysis. Methods to inhibit hydrolysis include:surface chemical modification,surface physical coating.
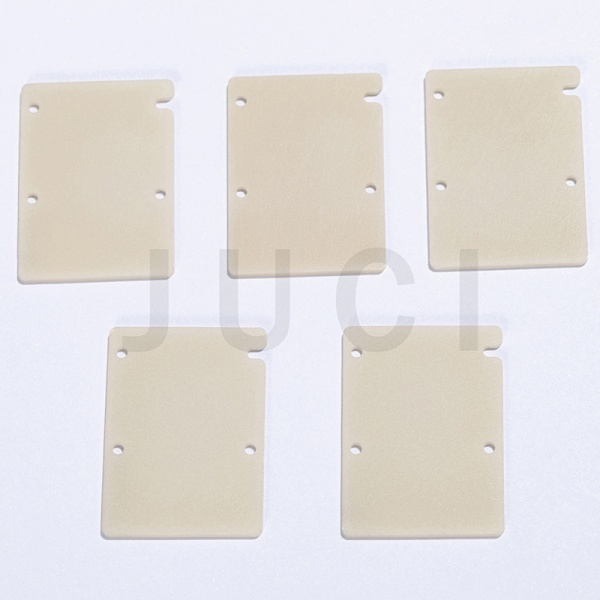
2. Substrate Fabrication
(1) Ceramic Substrate Forming
The primary method for preparing AlN ceramic substrates is tape casting.
(2) Critical Step—Sintering
Sintering is a crucial step in AlN substrate preparation.Pressureless sintering is the most common for AlN substrates due to its simplicity and low cost, but it typically requires high temperatures and sintering aids to achieve high performance.
The uniformity of sintering temperature in the furnace significantly impacts AlN ceramics. Research into temperature uniformity supports mass production, cost reduction, and commercialization.
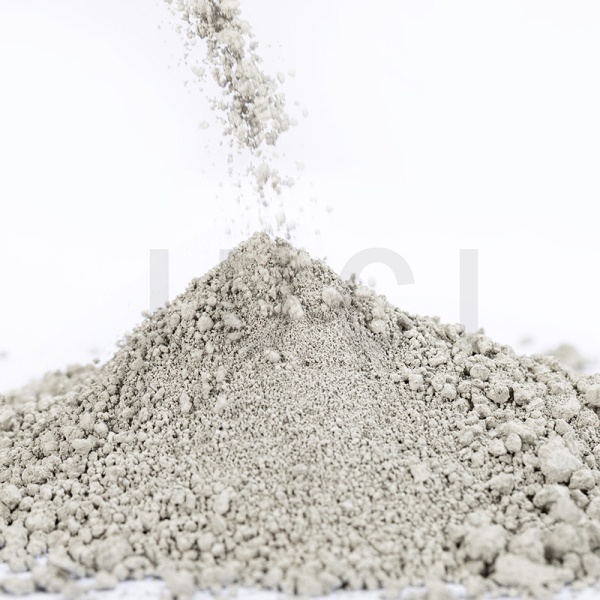
For dense ceramic sintering, adding sintering aids is the most economical and effective method.These materials serve two purposes during sintering:
They react with surface Al₂O₃ to form liquid aluminates, promoting mass transfer via viscous flow. This adjusts particle contact angles, fills pores, and enhances densification.They react with oxygen, reducing lattice oxygen content.
In reducing atmospheres, sintering time and temperature must be carefully controlled to prevent AlN reduction. Neutral atmospheres (e.g., N₂) avoid this issue, making them preferable for producing high-performance AlN ceramics.
About Xiamen Juci Technology Co., Ltd.
Xiamen Juci Technology Co., Ltd. is a high-tech enterprise specializing in the research, development, production, and sales of high-performance ceramic materials. As a leading AlN substrate manufacturer, the company is committed to providing high-quality aluminum nitride series products and solutions for industries such as electronics, semiconductors, and aerospace. With exceptional quality and service, Xiamen Juci has earned widespread trust from global customers.
Media Contact:
Xiamen Juci Technology Co., Ltd.
Phone: +86 592 7080230
Email: miki_huang@chinajuci.com
Website: www.jucialnglobal.com